Main Content
Research focus: Cellular responses to osmotic stress
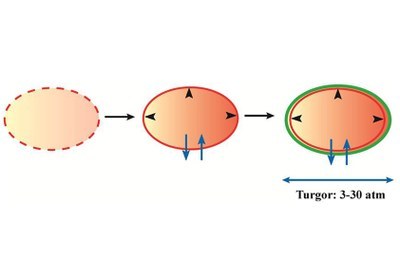
A key event in the evolution of primordial cells on earth was the development of the cytoplasmic membrane since it created a protected reaction chamber for the performance of life’s vital attributes (faithful copying of the genetic material and cell division, development of an ordered metabolism, generation of energy producing systems to fuel import and export transport processes). However, the invention of the semi-permeable cytoplasmic membrane and the subsequent development of a protective cell wall (e.g., the peptidoglycan sacculus) also created a severe problem for the microbial cells because this protected environment with its high concentrations of nucleic acids, proteins, organic metabolites and inorganic ions possesses a very substantial osmotic potential. This, in turn, creates an osmotic driving force that triggers water influx into the cell and thereby generates an intracellular hydrostatic pressure, the turgor, whose magnitude can in certain groups of microorganisms (e.g. Bacilli and Staphylococci) vastly exceed that present in a car tire. Turgor is considered essential for cell expansion and viability, but its magnitude is affected by fluctuations in the osmotic conditions of the varied habitats of microorganism.
Fig. 1. From a proto-cell to modern-day microbial cells: water-management and control of turgor is critical for cellular survival.

Microorganisms never developed the ability to actively pump water in or out of the cell to compensate for water fluxes across their cytoplasmic membrane that are elicited by variations in the external osmolarity. Instead, microorganisms learned in the course of evolution to determine the extent of cellular hydration and magnitude of turgor indirectly by dynamically modulating the osmotic potential of their cytoplasm in a direct response to cues emanating from osmotic changes in the environment. When they face hyperosmotic environments, they expel ions and organic compounds through the transient opening of mechanosensitive channels to prevent cell rupture. When they face hyperosmotic growth conditions they escape cellular dehydration and collapse of turgor by amassing ions (e.g., potassium) and a selected class of organic osmolytes, the so-called compatible solutes. Compatible solutes can be amassed by the microbial cells either through synthesis or through uptake in a direct response to increases in the osmolarity of their habitat.
Fig 2. Raster electron micrograph of the soil bacterium Bacillus subtilis (picture kindly provided by Laura Czech and Dr. Karl-Heinz Rexer, Philipps-Universität Marburg)

We are studying the genetic regulatory circuits that permit microbial cells to detect osmotic changes in their environment and study the cellular response systems that allow cells challenged by high salinity to cope with osmotic stress. We use the Gram-positive soil bacterium Bacillus subtilis and various taxonomically closely related Bacillus species as our primary model system. Important compatible solutes for Bacilli are the amino acid L-proline, the trimethylammonium compound glycine betaine and the tetrahydropyrimidine ectoine and its derivative hydroxyectoine. These compounds can either be synthesized in response to osmotic stress or can be taken up from the environment through osmotically controlled high-affinity uptake systems. Exposure of B. subtilis to high salinity (equivalent to dried-out soil) triggers rapid water efflux that is counteracted by the cell through increased uptake of potassium ions and the subsequent synthesis and import of compatible solutes (e.g., L-proline and glycine betaine). Compatible solute accumulation allows potassium efflux through dedicated extrusion systems and thereby permits the cell to reduce the ion strength of the cytoplasm without compromising its osmotic potential and ability to maintain physiological adequate levels of turgor. An important aspect of our work on the use of compatible solutes by B. subtilis as osmo-stress and temperature stress protectants is the analysis of osmotically controlled transport systems for the import of this class of compounds.We study the genetic regulation of the structural genes for these import systems in response to increased salinity (“osmoregulation”) and characterize structurally and biochemically components - primarily the extracellular ligand binding proteins - of ABC-transporters for various types of compatible solutes.
Fig. 3. Central osmo-stress response systems for the water-management by the soil Bacillus subtilis facing a high salinity environment.
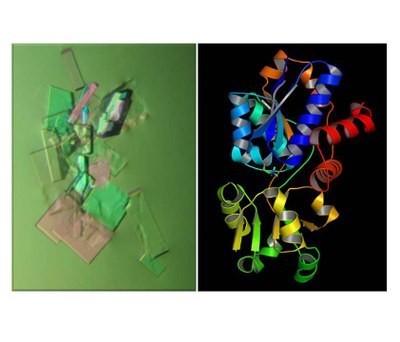
The soil bacterium Bacillus subtilis synthesizes the compatible solute glycine betaine through the oxidation of the precursor molecule choline. It imports for this purpose choline with high affinity through the osmotically inducible ABC transporter OpuB. Left: A view from the crystallization screen with the purified OpuBC protein (picture kindly provided by Dr. Sander H.J. Smith; University of Düsseldorf, Germany). Right: Overall crystal structure of the OpuBC protein in complex with its ligand choline (PDB code: 3R6U). The OpuBC crystal structure and the molecular determinants of the choline-binding site were report by Pittelkow et al. (J. Mol. Biol. 411:53-67, 2011).
Fig. 4. Structural analysis of the choline-binding protein OpuBC required for the functioning of the choline-specific OpuB ABC-transporter from Bacillus subtilis.

Our laboratory actively participates within the framework of the LOEWE initiative of the state of Hessen in the Centre for Synthetic Microbiology (SYMIKRO). Building on our extensive expertise in field of microbial stress resistance against extremes in osmolarity and growth temperature through uptake and synthesis of compatible solutes, we design portable genetic systems for transport and uptake systems for these compounds whose expression can be triggered at will. We assemble also genetic building blocks (“bio-bricks”) for the synthesis of L-proline and ectoine/hydroxyectoine from various microorganisms and synthetic gene constructs to streamline and maximize the high-level cellular production of these compounds for biotechnological purposes. Compatible solutes, also referred to in the literature as “chemical chaperones”, not only confer superior cellular resistance to osmotic stress but are also very efficient protectants against extremes in growth temperature; both low and high! Their ecophysiological relevance in natural settings can therefore not be underestimated for providing stress resistance to microbial cells!