Main Content
Chromatin regulators
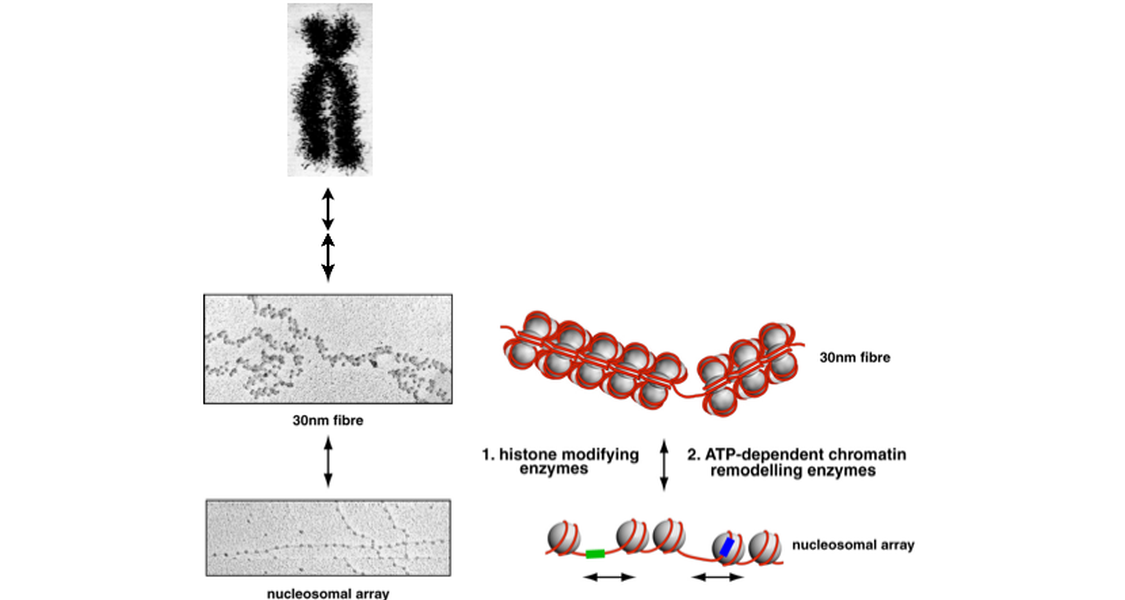
Eukaryotic genomes tend to be quite long. The 46 human chromosomes lined up end-to-end cover a length of about 2 metres. How on Earth can this amount of DNA fit into the tiny nucleus of a eukaryotic cell ? Well, the eukaryotic cell uses extremely sophisticated packaging material. The fundamental unit of this packaging material is the histone octamer, a complex composed of eight histone proteins. About 150 base pairs of double stranded DNA are wound around the octamer in 1.8 turns. The resulting structure (histone octamer plus DNA) is called a nucleosome. Nucleosomal arrays associate with other histone and non-histone proteins to compact the genetic material even further (the next level up is called the "30nm fibre") and to fold it into higher order structures. "Higher order structures" is the scientists way of saying: "We do not know what these structures look like but we know they must exist." The highest compaction level of a chromosome is reached during the metaphase of mitosis (the Barr body is another impressive example for extensive compaction of a chromosome) and can be visualized using a microscope.
The complex structure made up of DNA and its packaging materials is termed "chromatin" - different flavours of chromatin exist such as constitutive heterochromatin, facultative heterochromatin or euchromatin. They differ in compaction level and structure and roughly correspond to different activity states of the underlying DNA. Transcribed genes tend to localize to the more open euchromatin, silent genes are more often found in the more densely packed heterochromatin. Moreover, certain types of chromatin are characterized by the presence of particular histone modifications - histone hyperacetylation is associated with active chromatin, methylation at lysine 9 of histone H3 is a hallmark of heterochromatin etc.pp. However, no hard-and-fast rules seem to exist and it is not trivial to precisely define a term such as "heterochromatin". In fact, it can be quite amusing to listen to two chromatin researchers arguing about what heterochromatin is and what it is not.
The packaging of DNA into chromatin is posing a major problem for many DNA-dependent processes such as transcription, recombination, replication and repair. How on Earth can, say, a transcription factor gain access to its binding site on DNA when it is wrapped around histone octamers and the resulting nucleosomes are somewhere buried in still higher order structures. Fortunately, the eukaryotic cell not only possesses extremely sophisticated packaging material but also extremely sophisticated unpackaging and repackaging machines.
These machines are enzyme complexes that can use one of two strategies to locally or more globally change the packaging state of the genome. The ATP-dependent chromatin remodelers utilise the energy derived from ATP hydrolysis to actively change the association of DNA with histones and non-histone proteins, thus, unpacking DNA to some extent and making it more accessible for DNA binding factors. Some ATP-dependent chromatin remodelers seem to work in the reverse direction by helping to package DNA more efficiently into nucleosomes and higher order structure.
The second strategy is to post-translationally modify the packaging material, the histones, and so indirectly affect the association of DNA and histone molecules and chromatin structure. Many enzyme complexes that can modify histones have been identified. They include histone acetyltransferases, histone deacetylases, histone methyltransferases, histone demethylases, histone kinases and so on. These enzymes lay down the "histone code". According to the histone code hypothesis the presence of particular histone modifications (and their combinations) in a chromosomal region will determine chromatin structure, DNA accessibility and ultimately gene activity. "Tell me what histone modifications you have and I tell you how active you are." That's the idea.
But how do histone modifications translate into changes in chromatin structure ? This is one of the most fascinating questions in the field. There are several examples of chromatin associated proteins which possess domains which specifically bind to a particular histone modification. For example, the chromodomain of heterochromatic protein 1 (HP1) binds to histone H3 methylated at lysine residue K9 (H3K9me). Both HP1 and H3K9me are concentrated in heterochromatic regions of the genome. Another example is provided by the bromodomains - bromodomains bind acetylated histones and proteins that carry them tend to localize to active, hyperacetylated regions of the genome.
It appears that the "histone code" is "read" by these proteins but this does not answer the question, it transforms it into another one: How do the proteins that bind to modified histones change chromatin structure? Answer: No idea. Well, we have a few ideas but we do not yet have really good answers. That's why we are here.